A cut down version of this post appeared in the December 2022 edition of Lowflying. This full version is a little more wordy but contains more detail, images and with hyperlinks.
Putting the EV in sEVen – Part 2 : Conversion Tradeoffs
With my sEVen conversion car now purchased, I’m well and truly getting my feet wet on this project. There’s lots to be thought through still and I spend a lot of time talking to, and learning from, people who know more than me.
However, this has focussed my mind on what needs to be juggled when finalising the conversion. There are tradeoffs and compromises to be made and I thought it would be useful to go through some of the bigger ones here before we dive deeper into more detail in later articles.
I’ve broken the compromises down to a few categories. I could have started with just cost, time and performance but I think a little more detail would be more appropriate for this article.
Regulatory Backdrop
Before going too far into the weeds, it’s worth mentioning that everything has to be considered under the back drop of the prevailing regulations.
As we all know, to be road legal, a car older than 3 years has to have a valid MOT. At the moment though, besides the obvious problem of where to stick the emissions probe there isn’t much that’s different between the MOT of an ICE (Internal Combustion Engine) car and an EV (Electric Vehicle).
However, once a car has been converted to an EV you are required to notify DVLA of a change of vehicle status. You send off your V5 with accompanying documentation and wait for an amended V5 to come back with an “electric” taxation class and an “electricity” fuel type.
But this is where things can start to get a bit tricky. There are some horror stories out there of people getting their V5 confiscated at this point.
The problem comes with the DVLA’s test for “Radically Altered Vehicles”, which is based around a points scoring system (see table). The points are added up and used to determine whether an IVA (Individual Vehicle Approval) test and possible Q-plate are going to be your route. If you score less than 8 points, have modified the chassis, or if “there’s evidence of 2 vehicles being welded together”, then you get a Q-plate and must pass an IVA test. Points are awarded if you’ve kept that component unchanged from its original specification.
Part | Points |
Chassis, monocoque bodyshell | 5 |
Suspension (front and back) – original | 2 |
Axles (both) – original | 2 |
Transmission – original | 2 |
Steering assembly – original | 2 |
Engine – original | 1 |
The points system is reasonably straight forward, except for the following statement: Your vehicle must have 8 or more points, 5 of these points must come from having the original or new and unmodified chassis, monocoque bodyshell or frame.
Some classic conversions have come unstuck on this point. DVLA have, in some instances, apparently been quite pedantic with what constitutes a “change to chassis, monocoque bodyshell or frame”. Even two holes in the bottom of the boot has been enough to fall foul of this test, meaning the owner of the conversion needs to perform an IVA.
The IVA itself costs a few hundred pounds, and so has an obvious monetary consequence. But for some older classics, performing an IVA on a car that was never designed to pass the modern test is perhaps a daunting prospect. Fortunately, it should be possible for most Sevens to pass once the steps laid out in Caterham’s build manual have been implemented, at least as long as there are no chassis changes. I emphasise this because some of the documents supplied by Caterham when normally presenting a kit, and used to waive certain IVA tests, might be considered invalid if the chassis has been altered, causing further testing to be needed. To my knowledge this has yet to be tested with a Seven going through an IVA after an EV conversion.
While we’re talking about regulations it should be noted that there are standards bodies working on test procedures for EVs. The most advanced of those appears to be the United Nations “Regulation 100” document (who knew they were interested in EV’s?). This document is primarily aimed at the certification of new EV models, not conversions. But there is some talk of whether similar (or even the same) tests should be carried out on conversion.
The R-100 tests have been proposed to make sure that not only the occupants of the car are safe in normal use but also when there’s an accident: when we have to consider both the occupants and the emergency services. Not only would it be a bit of a head scratcher for the fire service when they attend a crumpled and fizzing sEVen, but it could be very dangerous given the propensity for some battery technologies to ignite if exposed to oxygen. The fire service has a database of what to do with each car model in an emergency – where to slice the A/B/C pillars to get the roof off etc. EV’s would also have details on kill switches and interlocks that kill power to motors and batteries. Which begs the question, where’s the database entry for the sEVen you converted in your garage?
In another example of time and cost implications, the R-100 document requires a battery module to be load tested and withstand a force of 10 tonnes and a vibration of 28g. Along with water immersion tests these tests could induce significant costs to achieve compliance and could, in practice, wipe out the Fred-in-a-shed conversion projects, pushing conversions to specialist converters who can afford to certify their installations and amortise their costs over the many cars they convert. The jury’s out on R-100 but we’re watching it with bated breath.
The Tradeoffs
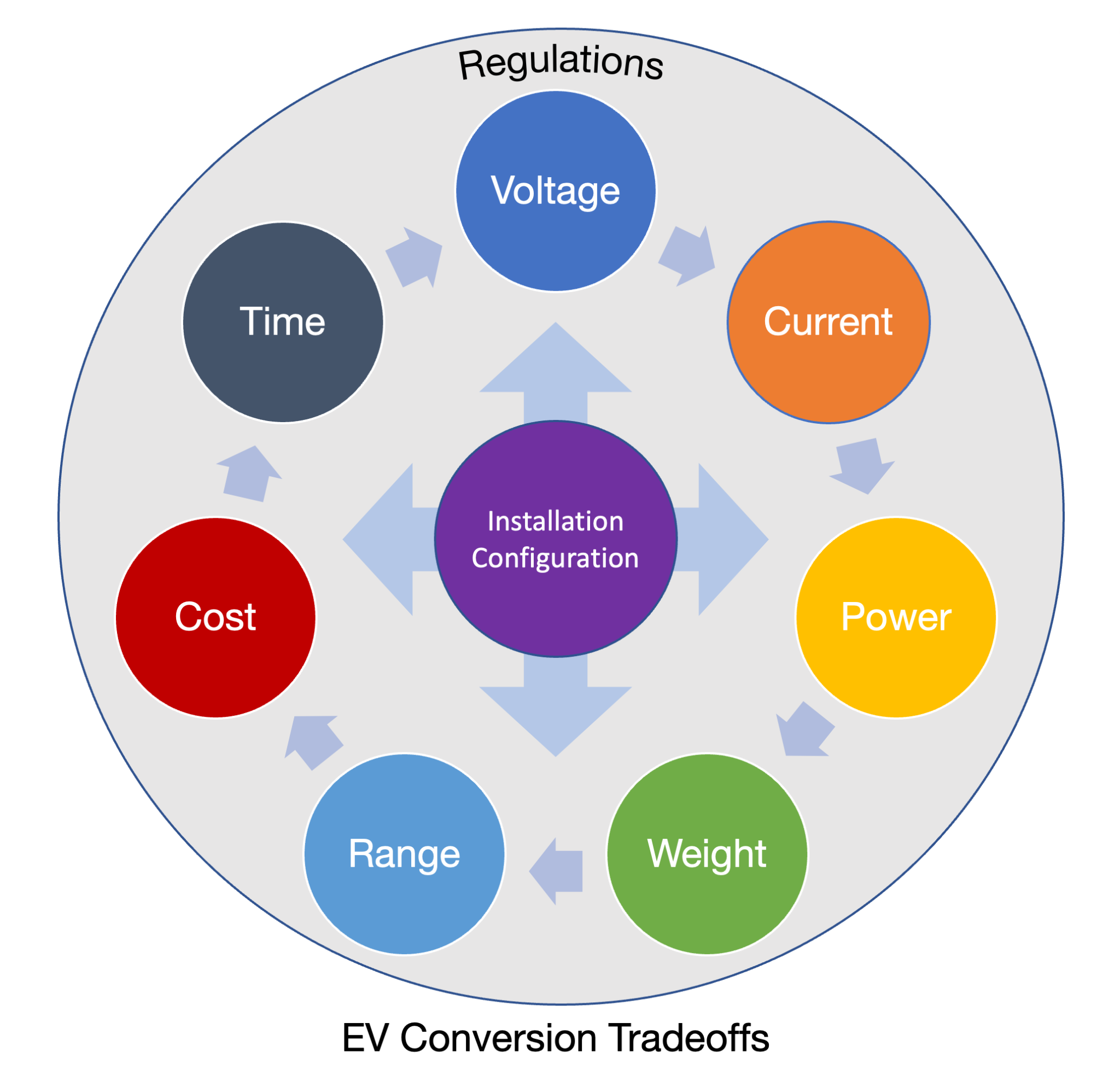
The following tradeoffs are often described as the major items that need to be juggled when considering an conversion. For some people and/or cars the decisions are simple: a matter of preference and personal circumstance. In other cases they all add to become a multi-dimensional conundrum to solve.
It’s also the case that these compromises are iterative. By which I mean you make some initial decisions and then move to looking at other tradeoffs, then realise your initial decisions don’t hold up to the new compromises and so you go round the loop again. You do this many times in the course of deciding on your design but it’s best to have solidified your thoughts long before you start to get physical with your car. Many converters will also use a lot of Computer Aided Design (CAD) at this stage – testing out ideas and seeing what might and might not work on a computer first.
There’s also a lot of spreadsheet work, calculating motor efficiencies for a given wheel diameter, differential ratios, possible reduction gearboxes, top speeds, acceleration, range and of course costs.
Installation Configuration
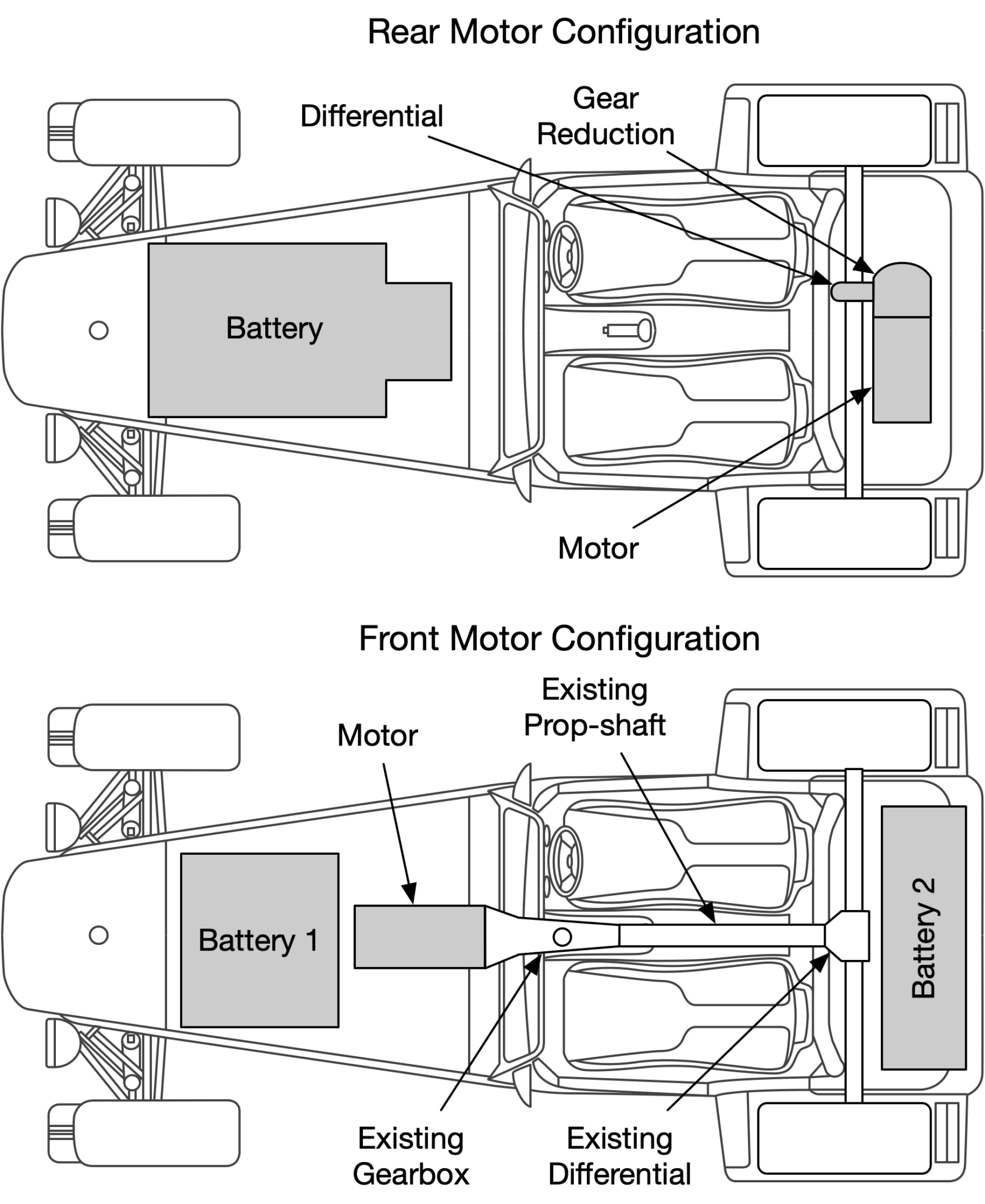
Whilst this tradeoff might not be as important as cost to many people, it is perhaps the most fundamental of all decisions. Should the motor be front or rear mounted? Should we keep the gearbox, clutch, prop-shaft and differential from the host car? Should we have a single motor, and differential, or two motors? What about wheel mounted motors?
The front/rear decision is a fundamental that isn’t actually as clear cut as it might appear – not least because of possible chassis changes. A rear mounted motor would make for a lighter car, allowing you to remove the gearbox, prop-shaft and differential from the design. It also means you have a lot more room up-front for a single large battery enclosure. However, the De-Dion suspension found in most Sevens, leaves a lot less room to fit a motor there, which might lead to significant chassis changes and so falling foul of the DVLA’s points system.
Then, perhaps, one of the big configuration compromises is which motor to use. Some motors work better in some orientations (and many must be installed in a specific orientation so coolant pickups work correctly) and will therefore only fit in certain spaces. Some also come with dedicated gear reduction boxes and/or differentials that also need to be accommodated.
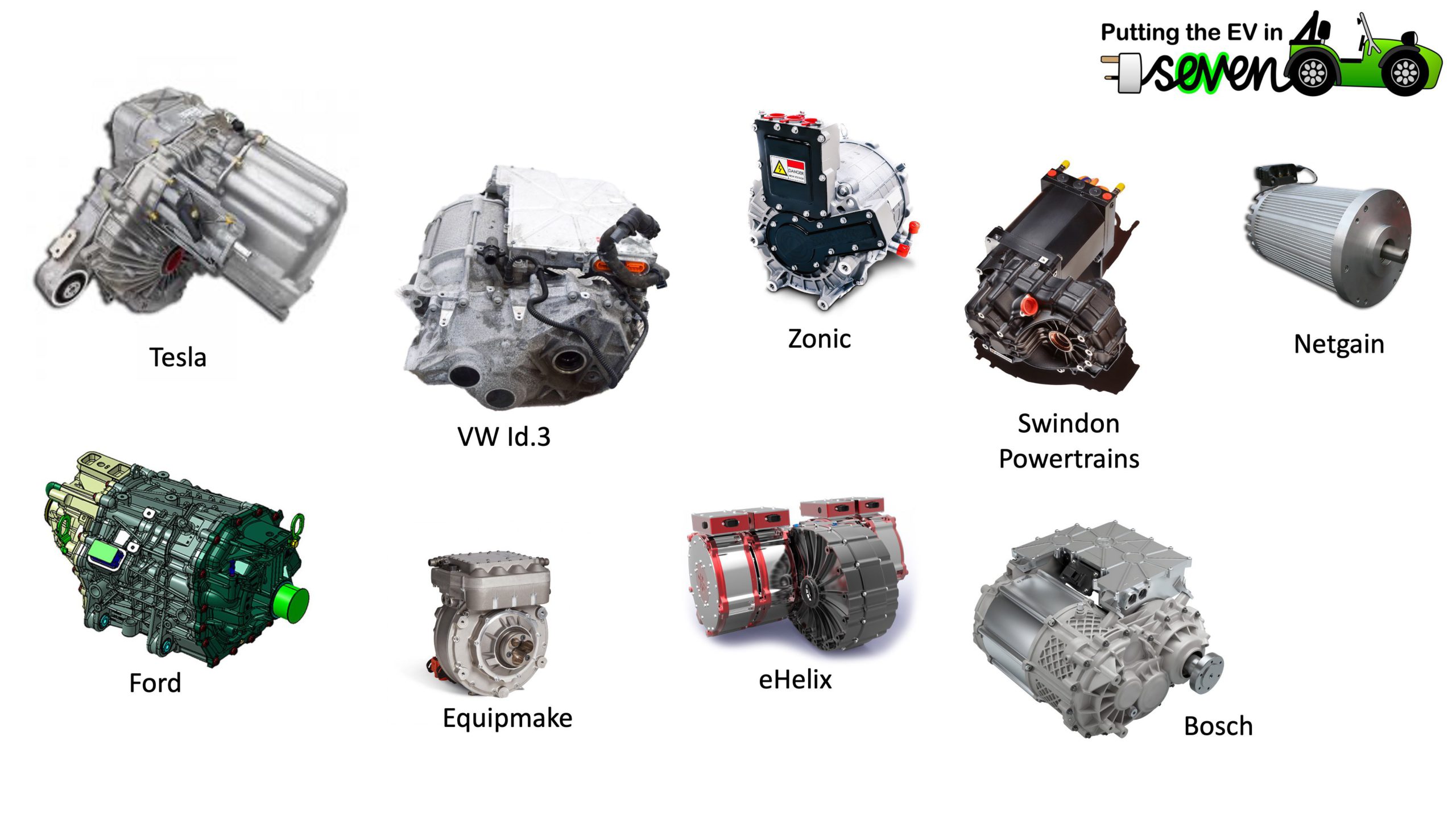
Other configuration compromises include, twin motors (for: no need for a differential, limited slip by default, against: increased software complexity, safety issues), wheel-motors (as with twin-motors, and for: possibly lower overall mass, might fit in a smaller space, against: increased unsprung mass), radial vs axial flux motors (axial may not need a gearbox and could easily be twinned for “no diff” installs, radial are much more widely available) and gearbox or not (for: some like the idea of swapping cogs, against: increased weight, need for clutch hydraulics, complexity).
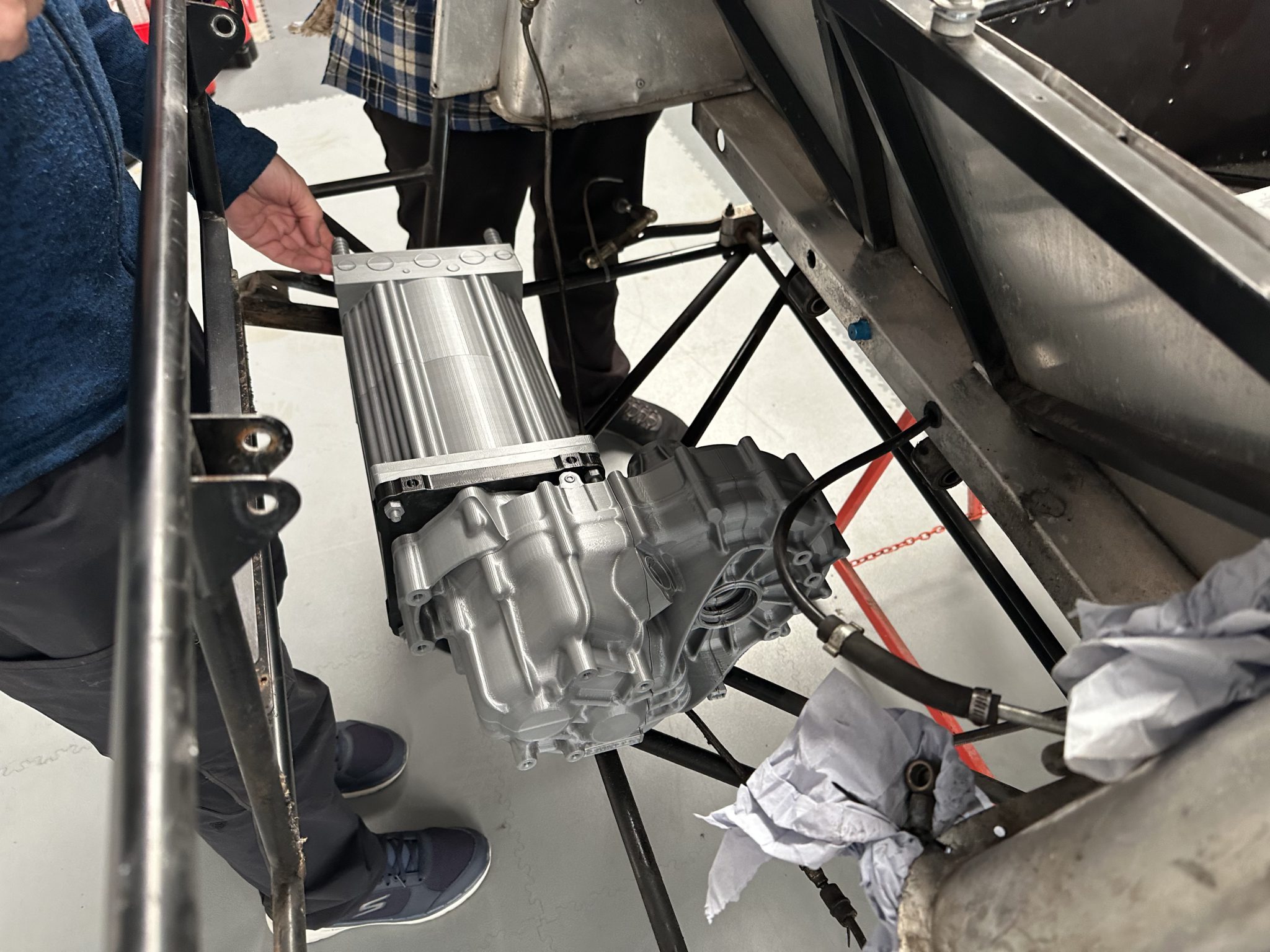
I spent a few hours with Simon Rogers at Meteor Motorsport a few weeks ago and an independent rear suspension (IRS) conversion might give more room in the rear for a motor mounted there. But of course, we’re back to more chassis changes again. Metoer Motorsport can be found here: Meteor Motorsport
These configuration decisions are one of the first for you to express an opinion on, but other factors may force you to go round the loop again.
Cost
This is probably the elephant in the room. There’s no getting away from it, EV conversions are currently expensive. Like all major Seven projects the sky’s the limit when it comes to writing cheques, but perhaps an EV conversion is THE most expensive thing you can do with a Seven. Conversion costs will start at a few 10’s of thousands of pounds and go rapidly up from there. The big expenses are the batteries and motor, with at least some hope that both will see reductions over the coming years, as tech improves and production quantities increase. But like any project, unless your pockets are very deep, it’s best to set a realistic budget and let other compromises take the hit.
Compromises with cost can mean: lower power, simpler installations keeping old components like gearboxes, reduced range, etc.
Voltage, Current and Power
These three tradeoffs are inextricably linked, but also considerably impact other tradeoffs and are a proxy for performance along with weight. As a reminder to those that don’t know, or have forgotten, the power of an electrical motor is defined as the product its voltage and current (P = I x V). If you have 100V applied to the motor and can deliver 100A, that gives 10kW of input power (I’m ignoring whether that’s input or output power at the moment). To put that into pounds, shillings and pence, there are 746W in a brake-horse-power (Bhp). So a 100kW motor is about 134bhp.
With that little bit of revision out of the way we can talk about some compromises, which come primarily from the batteries, though motor cooling tradeoffs and weight also relate to its power.
In terms of batteries though, realistically, the only battery technology that can be used at the moment is some form of Lithium-Ion (Li-Ion) battery – there are different chemistries floating about but freely available batteries are mostly all a form of Li-Ion. These are chemical batteries where charging moves Lithium atoms from one plate of the battery to the other. This stores charge in the battery which can be removed later. Li-Ion batteries have a typical voltage of around 3.6V per battery cell and so to power your car you need to put a lot of them in series, i.e. adding multiple 3.6V together until you get to the voltage your motor works best at.
The current (Amps) a battery can deliver, along with the voltage (Volts), determine the power your motor can use. You can increase the current delivered by your battery pack by putting battery cells in parallel. But, as well as the power we can deliver to the motor, the current capability of the battery pack will be a big determinant on the charging time. You can only charge a battery at a certain ratio of its maximum delivery current – known as its C rating. Decreasing your battery size to reduce range may therefore also affect your charging times.
So, we have many small Li-Ion cells connected in both series and in parallel to build up the voltage and current we want for our motor. Both of which can be varied to account for other compromises like range, weight and cost. Also, bear in mind that it’s the job of the on-board computers to manage those motor maximums as we drive the car. Some cars will have thousands of cells that go to make their battery packs.
We also need to consider heat. If we’re running at well below maximum power or for very short bursts at maximum, then we may get away with limited motor cooling. But if we’re looking at something a little more idiot-proof then we do need to consider motor cooling and probably battery cooling too. A typical modern motor runs at around 95% efficiency (often better if we’re running it at the right rpm and load) and if we apply that efficiency to a 200kW motor, that implies around 10kW’s of mostly heat losses that need to be dissipated. If you imagine 10, 1kW electric heaters under your bonnet, even they are still a lot less heat than generated by a Duratec – just ask a 420 owner how toasty their knees get on a hot summer’s day! But it’s still 10kW and that will cause overheating problems if we don’t cool the motor, which almost always come with internal plumbing already fitted just for this purpose. We might therefore need a coolant system with radiators, piping, pump, wiring etc. And depending on how long we run at an elevated power we may need a much more serious cooling system, along with a separate one for the batteries, with the compromises to find space for it all, and of course more weight.
Another twist to all of this comes in the interaction of voltage, battery state of charge and what’s called back-EMF (Electromotive Force).
With voltage applied, the motor takes current to generate torque and spins, as Michael Faraday discovered, due to the mutually perpendicular alignment of Current flow and Magnetic field. As he also discovered, if you spin a motor in a magnetic field it generates a back EMF in the stator windings of the opposite direction to the applied EMF.
The faster you spin the motor, the larger the Back EMF. Ultimately, if the applied DC voltage isn’t large enough compared to the Back EMF, then you can’t get more current into the motor to generate any more torque to and the Motor reaches its electrical speed limit.
This means that the decision of the DC Battery voltage is also dependent on the motor’s Back EMF characteristics, which are in turn dependent on its number of windings, its magnetic characteristics and the maximum RPM required of the motor, which is in turn dependent on the vehicle max-speed, gearing, tyre size etc. There’s a lot of if-this-then-that going on here.
The situation is also complicated by the fact that the battery voltage isn’t a constant, but reduces with Battery State of Charge (SOC). This means that if you want max rpm across the full SOC range, then the minimum battery voltage needs to be high enough compared to the maximum back EMF. OR you accept a degradation of performance as the battery charge falls-off.
There are ways around this that Faraday wouldn’t have even imagined! These involve complicated Digital Signal Processing (DSP) signal transform algorithms that use battery energy to dynamically reduce the magnetic field of the motor’s Permanent Magnets at high rpm. These techniques reduce the efficiency of the motor (you’re putting in energy to generate the new field) but also require not only a more sophisticated inverter to do the real time number crunching, but also a more powerful one where the power switching devices (FETs or IGBTs) need to be bigger to switch higher currents. This is called Field Weakening.
Thanks to an anonymous contributor for the suggested Back EMF twist! 👏🏻
Reaching the optimum voltage and current of the motor whilst still fitting all the batteries we want for our range, along with any cooling, is one of the big EV conversion tradeoffs.
Weight
Weight is a fairly simple tradeoff. If you want more power then you need a bigger motor, batteries and cooling. If you want more range then you need more batteries. Both of which equate directly to more weight (see the Range discussion for why more weight is a bad idea). Batteries are critical when it comes to any weight tradeoff.
Lets take a bit of a detour and talk about the specifics of batteries and weight for my project…
The Sigma SV “host” (some say “victim”) I’m using for my conversion currently weighs in at around 540kg as an ICE car, with almost no fuel in the tank and a minimal standard spec. From what I can find online it seems a Sigma engine, fuel tank, coolant and oil system are around half of that 540kg (I’m going to keep a lot of records of what all this weighs as I go through my project). So for a conversion to be weight neutral I need to be looking at a conversion component weight of around 270kg. The motor I’m looking at is about 60kg and the batteries about 190kg, not including battery box. Other components are going to be about 30kg. So on that measure I’m about the same. But in reality I think there’s going to be a pretty sizeable weight penalty in the conversion and if I can keep things below 650kg then I’ll be doing really well.
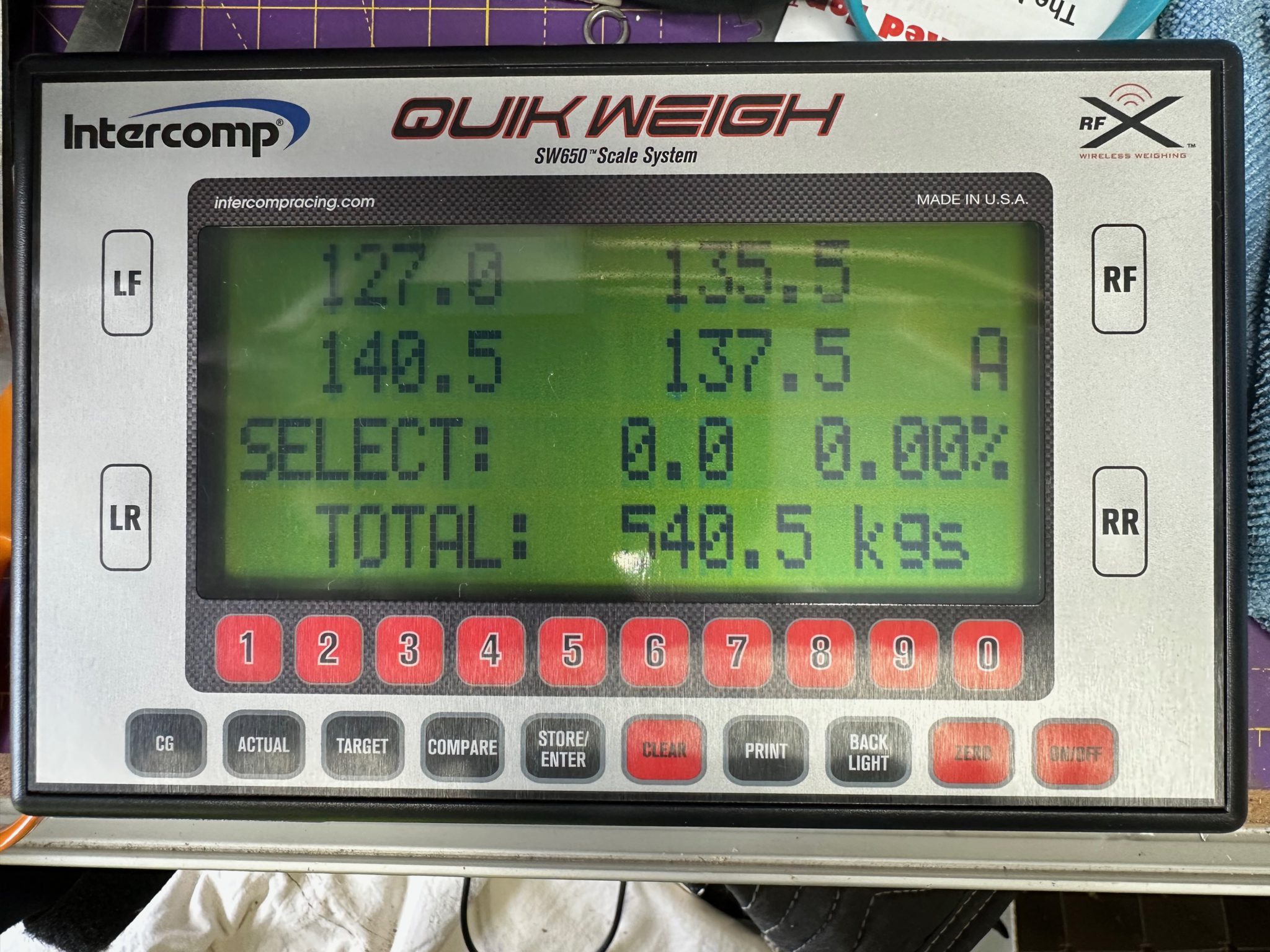
There are some compromises around the battery box(es) and weight as well though, so let’s look at an example of that.
For safety reasons the batteries need to be in a sturdy box. I bring this up under tradeoffs because the weight of the battery box(es) will depend on how well you can package them into the car. A simple rectangular cuboid battery box with a simple 6 sides gives the most efficient packaging. As soon as you start to split your battery install into multiple boxes, or convoluted shapes, then you increase the material needed to make the box. Let’s try and quantify that.
Typically I’ve seen conversions use 3mm sheet steel for the battery boxes, so lets assume that at the moment – there’s a whole debate we can have about the merits of thicker, less dense, less strong, aluminium vs thinner, more dense, stronger, steel, but we’ll leave that to another day. So a simple rectangular cuboid battery box for a 35kWh Li-Ion battery, gives a total volume of around 90L and would weigh around 30Kg (without any additional volume set aside for cooling). If we split this simple rectangular cuboid into two, each holding half the batteries, then each battery box weighs around 20Kg, making for a total of 40Kg for two boxes and an increase in 10Kg. This isn’t the end of the world though and maybe that might be a good tradeoff depending on other constraints, like range.
Range
As we all know with an ICE car, our mileage depends very much on how heavy our right foot is. And so, of course, that’s also the case for an EV.
We could get into some physics here, but I think we’ll not go down that avenue and I’ll just say that range is completely defined by how much energy you can carry and by how much energy gets lost making you go and slow. ICE cars are much less energy efficient than an EV, but can carry much more energy in a tank of unleaded.
This makes the job of an EV more tricky. But we can control a few parameters, like efficiency, weight and capacity.
Efficiency improvements can come from improving things like drag and electrical or mechanical losses. We’re not proposing to do a lot about drag in this project but I’ll come back to it later to illustrate just what a problem it is.
Electrical losses occur in all elements of an EV, the motor, cabling, voltage converters, etc.
For motors, they work best at a specific rpm and load, so getting them to run efficiently means gearing them correctly for the speeds you want to operate at. But additional gears add weight and potentially more mechanical losses. There’s also a choice of motor design that will affect its efficiency. Some motors are good at low RPM and others at high RPM. Tesla has a novel approach to motor design that uses a hybrid of two designs that offers the best of both worlds… but is too big to fit in a Seven.
Then there are other electrical losses. Electrical motors run on alternating current (AC) but a battery stores direct current (DC). Converting between the two, which is an intrinsic part of how an EV works, introduces electrical losses which manifest as heat. More efficient converters can increase range by reducing losses and technology improvements are helping here, so hopefully more efficient converters will come over time, but are probably more expensive. Similarly there are electrical losses in the cables moving all this power around the car. The high voltage (HV) cables in an EV have significant weight and keeping them thinner saves weight and cost. However, thinner cables have higher resistance and dissipate more energy as heat. There’s a direct compromise there.
One electrical efficiency, and range improvement, that has been used for many years on ICE cars but is especially applicable to an EV is regenerative breaking (regen). Regen takes kinetic energy (speed) and puts it back into the battery. It does this by turning the motor into a generator when you want to slow down. Without regen you have to use abrasive friction (normal) brakes to slow – turning the speed energy of the car into lost heat energy of the breaks. Regen is obviously better than normal breaking as at least some of the energy is put back into the battery to be used later. Regen is something that comes mostly for free in an EV, because the components used to make you go are exactly what’s needed for regen to make you slow. While it comes for free in terms of components it’s not all good news. There a losses taking the energy from the battery and turning it into speed and the same is true in reverse when taking the speed energy and putting it back into the battery. Each process is about 95% efficient and so those round trip loses can be as high as 10% of your regen-able energy. This means it’s still more efficient to drive an EV without relying on regen, driving smoothly without the need to slow down abruptly will still increase your range and is why EV drivers often turn off regen on the motorway.
While we’re talking about range, let’s talk about the real killer for current EV’s – drag. It also destroys your ICE range but because you don’t fill up so often with an ICE car I don’t think an average motorist thinks it’s such a big deal. But, in an EV you become very aware of the fact that speed kills your range. This is due to a square law that’s going on here. Drag losses are proportional to the square of speed and are the biggest losses when travelling quickly. To demonstrate this: if we increase our speed from 50mph to 60mph we increase the drag losses by 44%. Which shows you can lose over 1/4 of your range by going just 10mph faster.
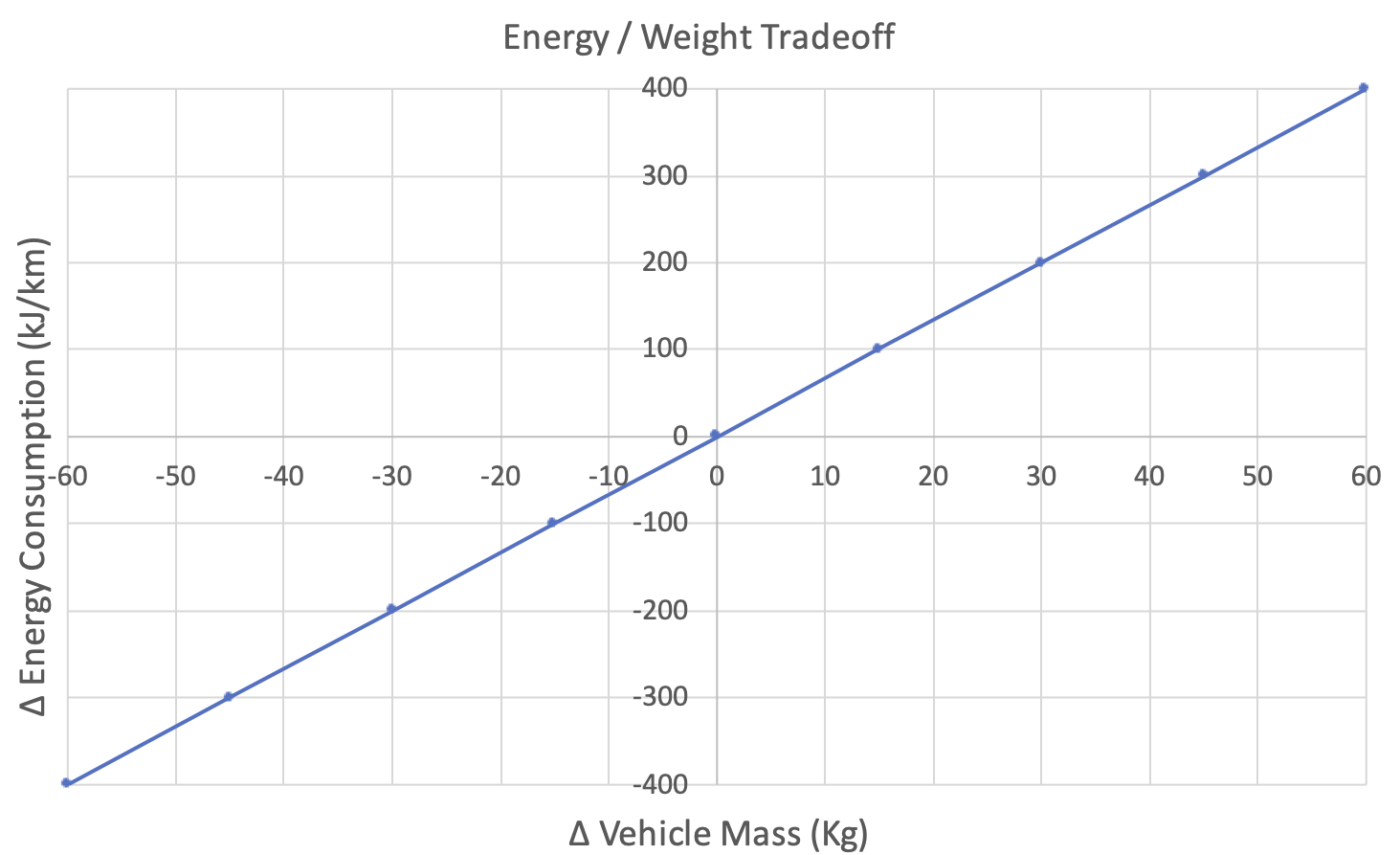
There’s also the question of weight and range. We’ve talked about how kinetic energy is gained and lost as we speed up and slow down. Well, kinetic energy is proportional to weight, and if you have losses (drag for instance) then the absolute losses will be higher for a higher weight, because it took you more energy to get to that speed. (Note from the future – this statement is a bit handwavey and I need a better example of why a heavier car is worse). There have been studies performed which show that for every 100Kg of weight saved, you improve energy consumption by around 15kJ/km (kilo-joules per kilometre). That would translate into a 5% saving going from 700Kg to 600Kg in a Seven. That’s not a huge amount and may be a compromise worth paying for more power or range, but of course might cause reduced handling performance that needs to be factored in too.
Time
Finally we come to the age old project management tradeoffs. You can pick any two from fast, cheap and good, you just can’t have all three!
For me though, I’m all about fast and specifically the aphorism – fail fast! In my experience you get a better, cheaper product more quickly if you “just do it” and learn from your mistake as you iterate. But of course you have to build those iterations into your plan too.
My project is an experiment, and because of the statements above, it’s just phase 1 of a bigger program. I’m not expecting to get something that’s perfect on the first go and in fact trying to do so is probably a fools errand, at least for a novice like me. Product development is all about learning from your mistakes early on when it’s cheaper to make those mistakes. If you try to come up with a finished contraption at your first attempt then you’ll still make mistakes and it will be more expensive to fix them. So, my aim is to get something going and then iterate. Once I have something on the road then switching batteries or motor, for instance, becomes just another iteration sub-project.
Conclusion
We’ve looked at a few of the major tradeoffs when thinking about a sEVen. It’s a mixture of mechanical, electrical, electronics, software, signal processing and project management skills. You need to be accomplished in all these areas to not fall foul of the compromises, and keep all of them in your head as you refine your design.
The trick to designing a sEVen is to pick a motor, matched battery with optimum voltage and current for best performance, that you can charge in the time you want, hit your weight and range goals, package it in the space you have, dissipate the heat, afford to pay for it, build it in a time frame that means your project is still relevant and at the end come up with something that DVLA will be happy with. Simples!
Leave a Comment